Speaker:
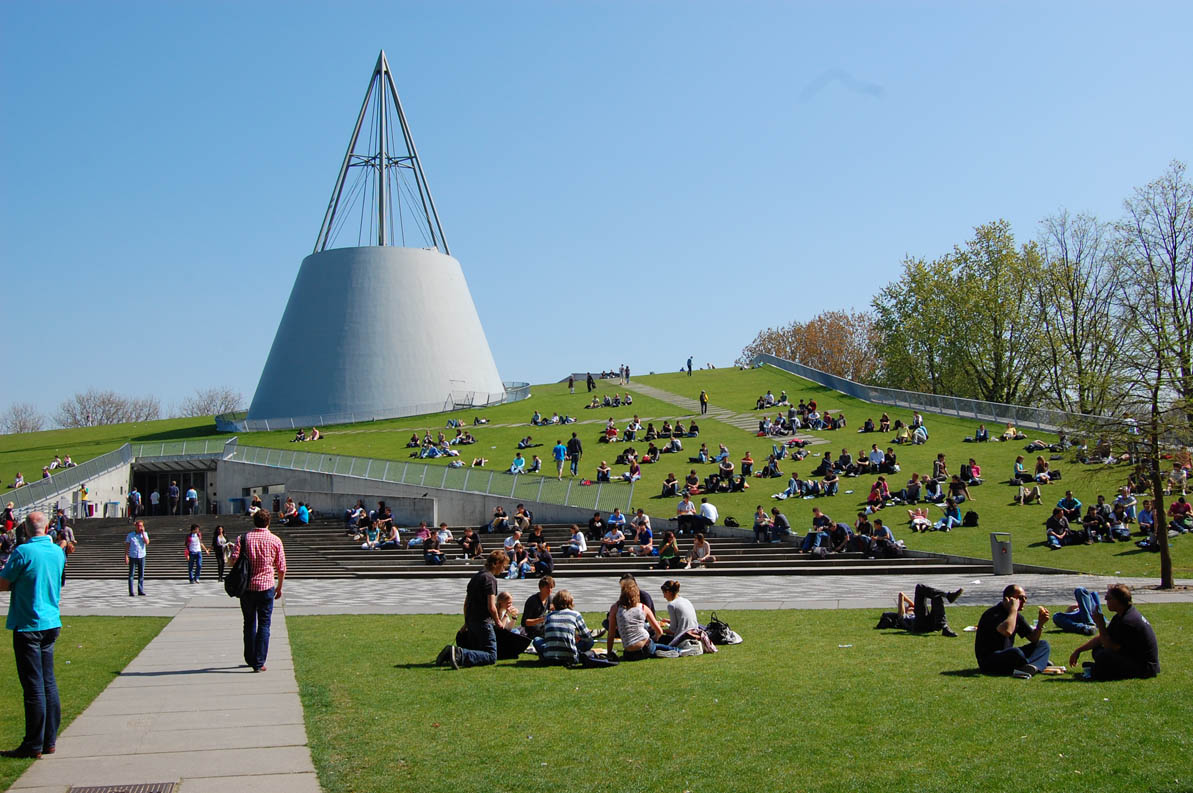
On June 27 ERL welcomes five researchers from Delft University of Technology (TU Delft) in the Netherlands to share their work on earth and atmospheric sciences:
Mr. Pieter Smets M.Sc.: "The study of sudden stratospheric warmings using infrasound"
Mr. Gil Averbuch M.Sc.: "Simulating anomalous acoustic transparency between ocean and atmosphere using the Fast Field Program"
Prof. Deyan Draganov: "Imaging subsurface structures using reflections retrieved from seismic interferometry with sources of opportunity"
Prof. Kees Weemstra: "Application of seismic interferometry by multidimensional deconvolution to ambient seismic noise recorded in Malargüe, Argentina"
Prof. Kees Wapenaar: "A single-sided representation for passive and active Green's function retrieval, time-reversal acoustics and holographic imaging"
The five presentations will last about 15 minutes each, with time for questions, starting 10:15am in 54-209.
Please RSVP to Dr. Niels Grobbe, ngrobbe@mit.edu.
The study of sudden stratospheric warmings using infrasound
Mr. Pieter Smets, M.Sc.
Infrasound has a long history for monitoring sudden stratospheric warmings. Several pioneering studies have focused on the various effects of a major warming on the propagation of infrasound, described throughout this chapter. A clear transition has been made from observing anomalous signatures towards the use of these signals to study anomalies in upper atmospheric conditions. First studies describe the various infrasonic signatures of a major warming. In general, the significant change in observed infrasound characteristics correspond to summer-like conditions in midwinter. More subtle changes occur during a minor warming, recognisable by the presence of a bidirectional stratospheric duct. A combined analysis of all signal characteristics unravels the general stratospheric structure throughout the life cycle of the warming. Then, infrasound observations are used to evaluate the state of the atmosphere as represented by various NWP models. A new methodology, comparing regional volcano infrasound with simulations using various forecast steps, indicates interesting variations in stratospheric forecast skill.
Simulating anomalous acoustic transparency between ocean and atmosphere using the Fast Field Program
Mr. Gil Averbuch, M.Sc.
A seismo-acoustic event is an event in which seismic energy transfers to acoustic energy in the oceans and/or atmosphere (infrasound waves) and vice versa. Ray theory predicts that less than 0:01% of the energy will be transmitted through the earth/ocean into the atmosphere. Therefore, it is usually considered as a perfect reflector. Clear observations of infrasound waves that originated from underwater or underground sources contradict
the classical theory. Under specific conditions, anomalous transparency of the ocean-atmosphere interface is predicted. It allows most of the energy to be transmitted into the atmosphere and more horizontal propagating waves will appear. In this presentation we will limit ourselves to the acoustic case only. Acoustic coupling through ocean-atmosphere interface is simulated using the Fast Field Program (FFP). Numerical results will be presented.
Imaging subsurface structures using reflections retrieved from seismic interferometry with sources of opportunity
Prof.dr.ir. Deyan Draganov
(presenting work by D. Draganov, B. Boullenger, S. Minato, K. Wapenaar, J. Thorbecke, E. Ruigrok (Utrecht U.), C. Rowe (Los Alamos), B. Paap (TNO), A. Verdel (TNO), M. Gomez (CNEA))
The reflection seismic method is the most frequently used exploration method for imaging and monitoring subsurface structures with high resolution. It has proven its qualities from the scale of regional seismology to the scale of near-surface applications that look just a few meters below the surface. The reflection method uses controlled active sources at known positions to give rise to reflections recorded at known receiver positions. The reflections’ two-wave traveltime is used to extract desired information about and image the subsurface structures. When active sources are unavailable or undesired, one can retrieve body-wave reflections from application of seismic interferometry (SI) to sources of opportunity – quakes, tremors, ambient noise, or even man-made sources not connected to the exploration campaign. We show examples of imaging of subsurface structures using reflections retrieved from quakes and ambient noise. We apply SI by autocorrelation to global earthquake to image seismic and aseismic parts of the Nazca plate and the Moho at these places, SI by multidimensional deconvolution to P-wave coda from local earthquakes to image the Moho and the crust at the same places, and SI by autocorrelation to deep moonquakes to image the lunar Moho and to ambient noise to monitor CO2 sequestration.
Application of seismic interferometry by multidimensional deconvolution to ambient seismic noise recorded in Malargüe, Argentina
Prof.dr. Kees Weemstra
(Presenting work by D. Draganov, E. Ruigrok, J. Hunziker, M. Gomez, K. Wapenaar)
Obtaining new seismic responses from existing recordings is generally referred to as seismic interferometry (SI). Conventionally, these seismic interferometric responses are retrieved by simple crosscorrelation of recordings made by separate receivers: a first receiver acts as a 'virtual source' whose response is retrieved at the other receivers. When surface waves are retrieved, the newly retrieved responses can be used to extract receiver-receiver phase velocities. These phase velocities often serve as input parameters for tomographic inverse problems. Another application of SI exploits the temporal stability of the multiply scattered arrivals (the coda). For all applications, however, the accuracy of the retrieved responses is paramount. In practice, this accuracy is often degraded by irregularities in the illumination pattern: correct response retrieval relies on a uniform illumination of the receivers. Reformulating the theory underlying seismic interferometry by crosscorrelation as a multidimensional deconvolution (MDD) process, allows for correction of these non-uniform illumination patterns by means of a so-called point-spread function (PSF). We apply SI by MDD to surface-wave data recorded by the Malargüe seismic array in western Argentina. The aperture of the array is approximately 60 km and it is located on a plateau just east of the Andean mountain range. The array has a T-shape, which makes it very well suited for the application of SI by MDD. We select time windows dominated by surface-wave noise traveling in a favorable direction, that is, traversing the line of virtual sources before arriving at the receivers at which we aim to retrieve the virtual-source responses. These time windows are selected based upon the slownesses along the two receiver lines. From the selected time windows, virtual-source responses are retrieved by computation of ensemble-averaged crosscorrelations. Similarly, ensemble-averaged crosscorrelations between the positions of the virtual sources are computed: the PSF. We use the PSF to deconvolve the effect of illumination irregularities and the source function from the virtual-source responses retrieved by crosscorrelation. The combined effect of time-window selection and MDD results in more accurate and temporally stable surface-wave responses.
A single-sided representation for passive and active Green's function retrieval, time-reversal acoustics and holographic imaging
Prof.dr.ir. Kees Wapenaar
The homogeneous Green's function, defined as the superposition of the Green's function and its time-reversal, plays an important role in a variety of acoustic applications, such as passive and active acoustic Green's function retrieval, seismic interferometry, time-reversal acoustics and holographic imaging. An exact representation of the homogeneous Green's function originates from the field of optical holographic imaging (Porter, 1970, JOSA). In this representation, the homogeneous Green's function between two points A and B is expressed as an integral along an arbitrary boundary enclosing A and B. This implies that the Green's function between A and B can be retrieved from measurements carried out at a closed boundary, or, via reciprocity, from passive observations at A and B of the responses to sources on a closed boundary. In practical situations, the closed-boundary integral usually needs to be approximated by an open-boundary integral. This can lead to significant artifacts in the retrieved Green's function. I will discuss a new, single-sided, representation of the homogeneous Green's function, which obviates the need for omnidirectional access. Like the classical closed-boundary representation, this new single-sided representation fully accounts for multiple scattering. I will indicate applications of this new representation in the aforementioned fields.